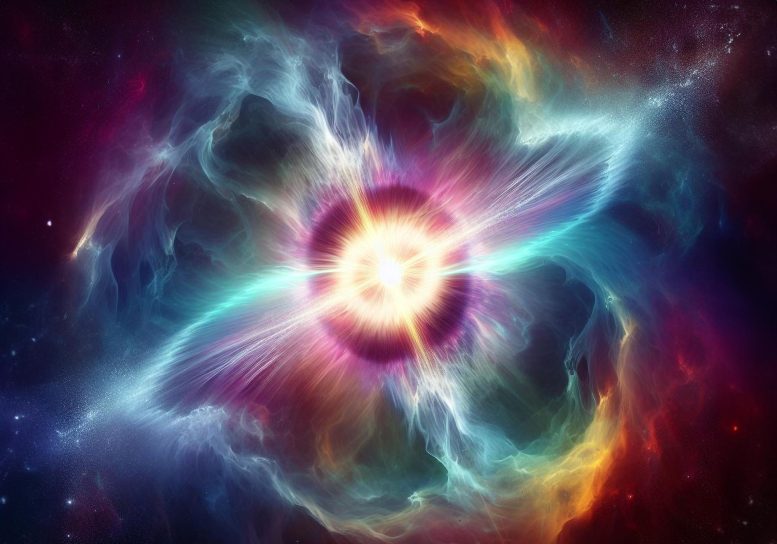
Researchers have linked the periodic pulses of neutron stars to internal defects influenced by superfluid vortices, with a new model suggesting that these defects follow a power-law pattern observed in various natural phenomena. Credit: SciTechDaily.com
A recent study has revealed the origin of the mysterious “heartbeats” observed in neutron stars, linking them to defects caused by the dynamics of superfluid vortices.
The researchers found that these defects follow a power-law distribution similar to other complex systems and developed a model based on quantum vortex networks that matches the observed data without additional tuning.
Discovering the heartbeat of neutron stars
The code that twinkles the stars in Netflix’s “3 Body Troubles” may be science fiction. However, a new study deciphered the erratic vibrations of neutron stars, revealing the twisted origins of the mysterious “heartbeats” of these dead stars.
When neutron stars—the ultra-dense remnants of massive stars that exploded in supernovae—were first discovered in 1967, astronomers thought their strange periodic pulses might be signals from an alien civilization. Although we now know that these “heartbeats” originate from stellar corpse radiation beams, not extraterrestrial life, their precision makes them excellent cosmic clocks for studying astrophysical phenomena, such as rotation rates and dynamics interior of the heavenly bodies.
Sometimes, however, their schedule ACCURATELY it is interrupted by pulses that inexplicably arrive earlier, signaling a glitch or a sudden acceleration in the neutron star’s spins. While their exact causes remain unclear, error energies have been observed to follow a power law (also known as a scaling law)—a mathematical relationship reflected in many complex systems from wealth inequality to frequency-magnitude patterns. in earthquakes. Just as smaller earthquakes occur more often than larger ones, low-energy faults are more common than high-energy ones in neutron stars.
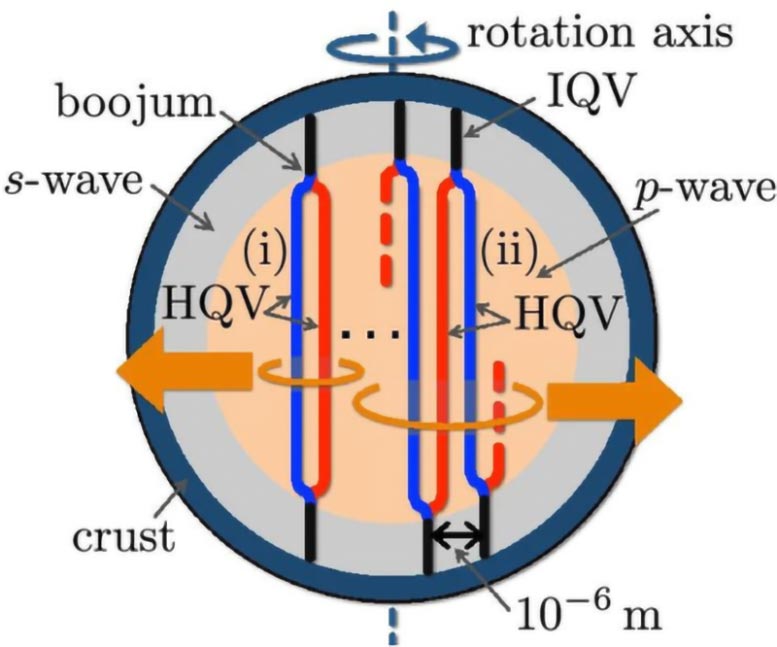
The image shows the quantum vortex network model proposed by the authors of the study. The inner core of the p wave (pink) surrounds the outer core of the S wave (grey). Credit: Muneto Nitta and Shigehiro Yasui
By reanalyzing 533 updated data sets from observations of rapidly spinning neutron stars, called pulsars, a team of physicists found that their proposed quantum vortex network matches naturally with calculations on the power-law behavior of energies of fault without the need for additional tuning. unlike past models. Their findings are published in the journal Scientific Reports.
Superfluid vortices take a new turn
“More than half a century has passed since the discovery of neutron stars, but the mechanism of why the defects occur is still not understood. So we proposed a model to explain this phenomenon,” said the study’s corresponding author Muneto Nitta, a specially appointed professor and co-investigator at Hiroshima University’s International Institute for Stability with Knotted Chiral Meta Matter (WPI-SKCM).2).
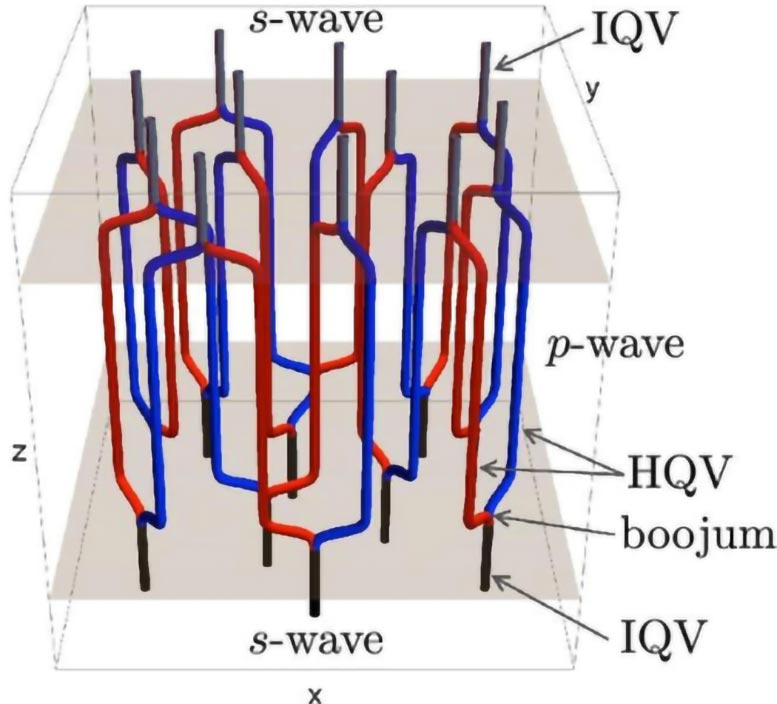
3D configuration of the quantum vortex network. Credit: Muneto Nitta and Shigehiro Yasui
Previous studies have proposed two main theories to explain these faults: starquakes and superfluid vortex avalanches. While stars, which behave like earthquakes, can explain the observed power-law pattern, they cannot account for all types of faults. Superfluid vortices are the widely invoked explanation.
“In the standard scenario, the researchers consider that the avalanche of unprotected vortices could explain the origin of the faults,” Nitta said.
However, there has not been a consensus on what can cause eddies to collapse catastrophically.
Key Insights into the Dynamics of Neutron Stars
“If there was no pinning, this would mean that the superfluid releases the vortices one at a time, allowing for a smooth adjustment in spin speed. There would be no avalanches and defects,” said Nitta.
“But in our case, we didn’t need any locking mechanism or additional parameters. We only needed to consider the structure of p- and s-wave superfluids. In this structure, all the vortices are connected to each other in each group, so they cannot be released one by one. Instead, neutron star it should release a large number of vortices at once. This is the key point of our model.”
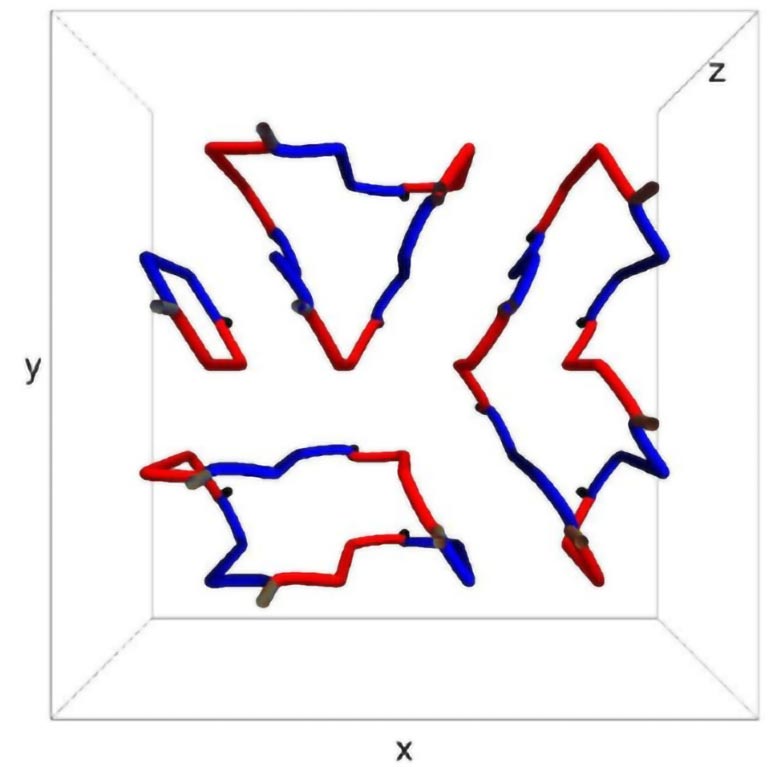
Top view of a quantum vortex network. Credit: Muneto Nitta and Shigehiro Yasui
As the superfluid core of a neutron star rotates at a constant rate, its ordinary component slows down its rotation by releasing gravitational waves and electromagnetic pulses. Over time, their velocity mismatch increases, so the star expels the superfluid vortices, which carry some of the angular momentum, to regain equilibrium. However, as the superfluid vortices are entangled, they drag others along, explaining the defects.
Twisted arrays and real-world data alignment
To explain how vortices form twisted clusters, the researchers proposed the existence of two types of superfluids in neutron stars. S-wave superfluidity, which dominates the relatively smooth environment of the outer core, supports the formation of integer-quantized vortices (IQVs). In contrast, the p -wave superfluidity that prevails in the extreme conditions of the inner core favors semi-quantized vortices (HQVs). As a result, each IQV in the s-wave outer core splits into two HQVs upon entering the p-wave inner core, forming a cactus-like superfluid structure known as a boojum. As more HQVs separate from IQVs and connect through boojums, the dynamics of vortex clusters become increasingly complex, much like cactus wings sprouting and intertwining with neighboring branches, creating intricate patterns.
The researchers performed simulations and found that the exponent for the power-law behavior of the defect energies in their model (0.8±0.2) closely matched the observed data (0.88±0.03). This shows that their proposed framework accurately reflects real-world neutron star defects.
“Our argument, although simple, is very powerful. Although we cannot directly observe the p-wave superfluid inside, the logical consequence of its existence is the power-law behavior of the cluster sizes obtained from the simulations. Translating this into a corresponding power-law distribution of error energies showed that it matches the observations,” said co-author Shigehiro Yasui, a postdoctoral researcher at WPI-SKCM.2 and Associate Professor at Nishogakusha University.
“A neutron star is a very special situation because the three fields of astrophysics, nuclear physics and condensed matter physics meet at one point. It is very difficult to observe directly because neutron stars exist so far away from us, therefore, we have to a deep connection between internal structure and some observational data from neutron stars.Marmorini, Shigehiro Yasui and Muneto Nitta, 3 Apr 2024, Scientific Reports.
DOI: 10.1038/s41598-024-56383-w
Yasui and Nitta are also affiliated with Keio University’s Department of Physics and Center for Natural Science Research and Education. Another collaborator on the study is Giacomo Marmorini from the Physics Departments of both Nihon University and Aoyama Gakuin University.